
CHU Lab @ CHEM-NTHU
Biophysics & Time-resolved Spectroscopy Laboratory


Instrumentation
Fluorescent temperature jump
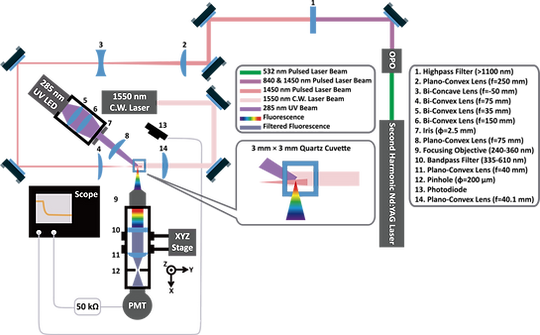
A continuous-wave 280-nm LED served as the probe beam to excite tryptophan. Pulsed infrared laser at 1450 nm was introduced to the sample compartment for rapidly heating the water. The fluorescence of tryptophan was collected with a microspot focusing objective and further filtered to define the detection wavelengths range of 335−430 nm. Sequentially, the fluorescence was focused through a pinhole of 100 μm diameter by a lens to achieve the small-volume detection. The temperature evolution, ΔT, was derived from the fluorescence evolution of the pure tryptophan,
ΔT= α^-1 (V0 - Vt) / V0
where V0 and Vt refer to the voltage outputs from the photomultiplier tube, which are proportional to the tryptophan fluorescence upon exposure at 280 nm, before and after introducing the infrared heating laser, respectively. α is 2% °C^−1 for the fluorescence decrement derived from the temperature-dependent tryptophan fluorescence.
Step-scan Fourier-transform interferometer
Absorption mode
The time-resolved infrared difference spectra were collected using a Fourier-transform infrared spectrometer (Vertex 80, Bruker) operated in the step-scan mode and the data were acquired using ac/dc coupling method. In the absence of laser pulse, the dc-coupled signal from the mercury cadmium telluride (MCT) detector was directly sent to the internal analog-to-digital convertor installed in Vertex 80 for the calculation of background spectrum and for the phase correction. In the presence of the laser excitation, the dc-coupled signal from the MCT detector was sent to a voltage amplifier, coupled by ac mode, further amplified. The output signal was recorded by an external ADC. After the completion of the data acquisition in the whole optical retardations, the time-evolved single channel spectra were obtained via the reverse Fourier transformation of the interferograms and the corresponding time-resolved difference spectra were calculated.
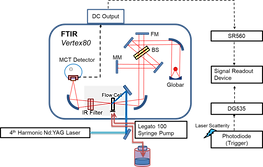
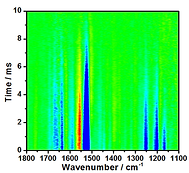
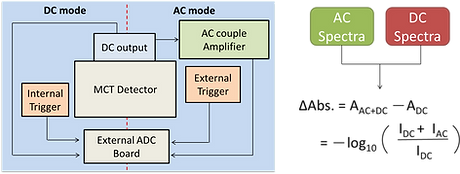
Emission mode
The dried sample on the CaF2 window was mounted at the focal point of the parabolic mirror for collecting the emission at the side of the interferometer. A set of sleeves was connected to the sample compartment and a small amount of NaOH was added to adsorb the H2O and CO2 to prevent as much of the spectral interference in the infrared optical path as possible. A frequency-doubled Nd:YAG laser provided 5-ns pulses at 532 nm for the excitation of the surface plasmonic resonance band of gold nanoparticles.
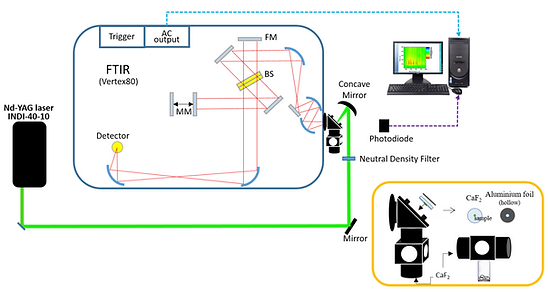
A photodiode was used to collect the scattering of the laser to serve as a trigger. Time-resolved IR emission spectra were collected using a FTIR operated in the step-scan mode. The raw interferograms were collected using the ac-coupled method. A mercury cadmium telluride detector was employed to collect the infrared emission and directly send it to an analog-to-digital convertor without further amplification and electronic filtering. No optical filter was positioned in the midst of the optical path. The spectral range was mainly defined by the detector and other optical components at 900–4,000 cm^–1. After the completion of the data acquisition in all the optical retardations, the time-evolved emission spectra were obtained via reverse FT of the interferograms.
Fluorescent thermometer
The experimental module included a continuous-wave 532-nm laser for exciting gold nanoparticles, continuous-wave 280-nm light for exciting tryptophan, fluorescence collecting optics, and a data acquisition system. The continuous-wave 532 nm diode laser provided power of 102 mWatt in a beam diameter of 0.83 mm (FWHM) to serve as the excitation source. The incident intensity of the 532-nm laser was adjustable by the neutral density filters and monitored with a power meter. The laser was introduced to a sample cell, a 2 mm x 2 mm (inner) square quartz tube.The 280 nm light was provided by a solar generator upon wavelength selection with a monochromator. The 280-nm beam passed through a 100-mm pinhole to define the probing cross section and propagated opposite to the 532-nm beam. At the center of the sample cell, 9 mm from the pinhole, the diameter of the 280-nm beam was 0.81 mm, slightly smaller than that of the 532-nm excitation beam (0.83 mm), to ascertain that the fluorescence intensity alteration originated from the heating volume upon excitation of the nanoparticles. The fluorescence of tryptophan was filtered by two edge filters at 450 nm to remove most of the scattering 532-nm light and a bandpass filter to define the detection wavelength in 300–450 nm in front of a photomultiplier. The electronic signal was recorded by an oscilloscope. The exposure period was controlled to 500 seconds using a mechanical shutter.
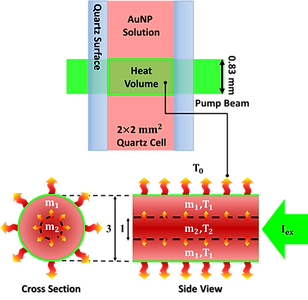

Transient absorption
Tunable pulsed laser
An optical parametric oscillator (basiScan-M/120/HE, Spectra-Physics) pumped by a frequency-tripled Nd:YAG laser (INDI-40-10, Spectra-Physics) provided a 532 nm pulse with a bandwidth of 3 ns. A mechanical shutter (VS25S2T1, Uniblitz) was employed to decouple the 10 Hz irradiation to 5, 1, 2, and 0.5 Hz. The laser
pulse was introduced via fiber optics (Model 76842, Newports) to the front of the sample cuvette.
Continuous probe
A dim broadband beam from the tungsten halogen lamp (DH-2000, Ocean Optics), perpendicular to the excitation laser, was employed for continuous probing, coupled with a monochromator (Model 218, McPherson) to define the optical modulation at 370−710 nm.
Data acquisition
Photon signals were collected by a photomultiplier tube, and the data were acquired with a 200 MHz oscilloscope. Evolution of the absorbance difference was derived by −ΔA = log(St/S0), where St and S0 represent the dc-coupled voltages in the presence and absence of the excitation laser, respectively.
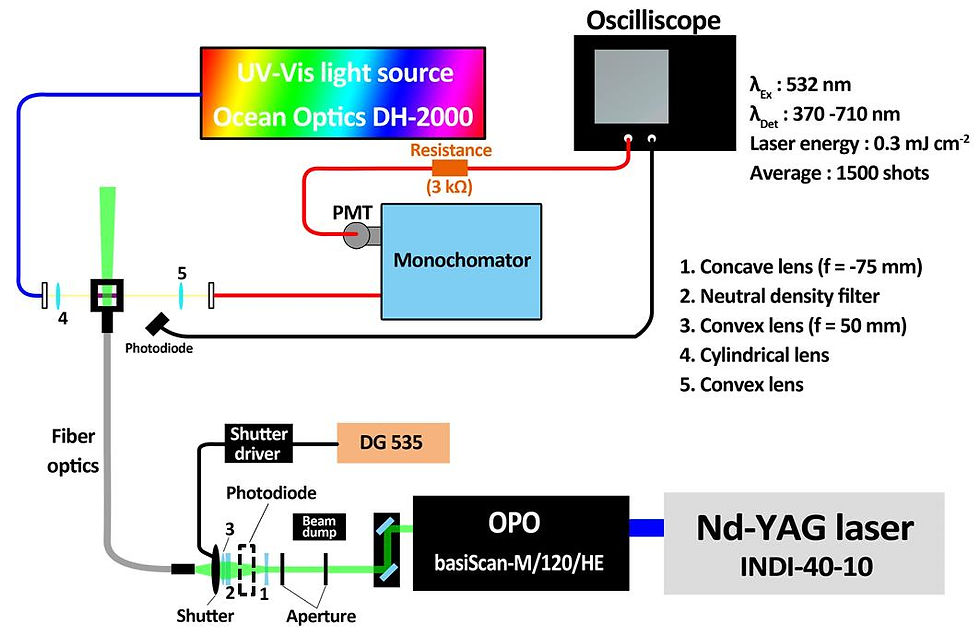
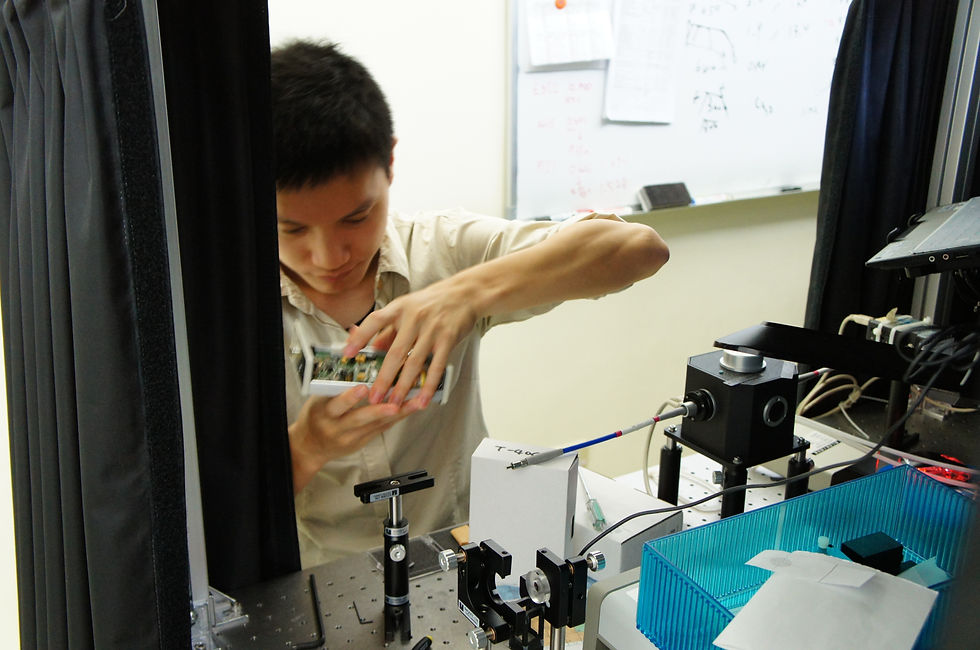

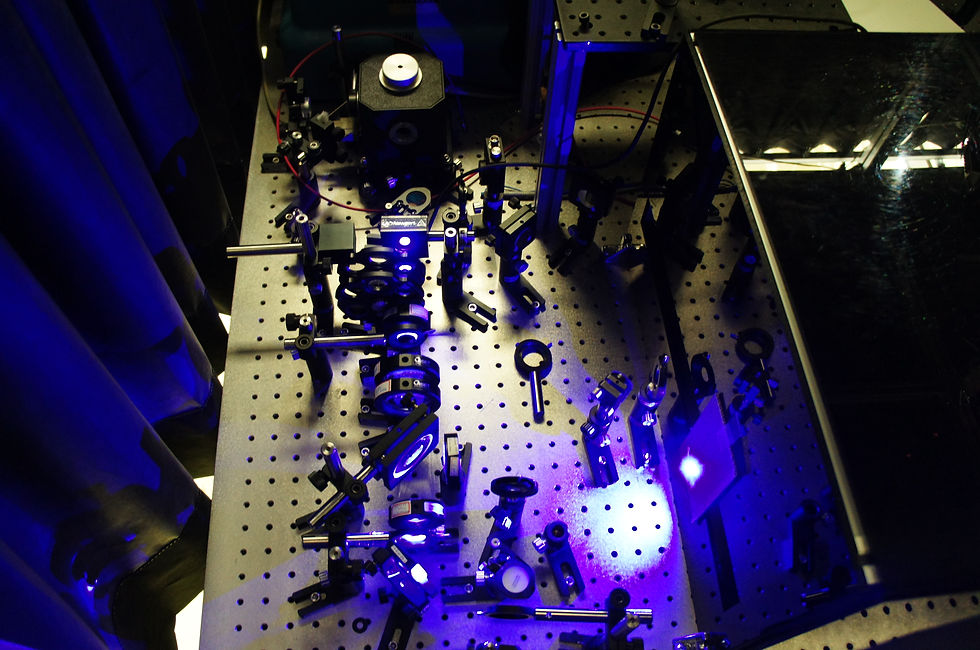

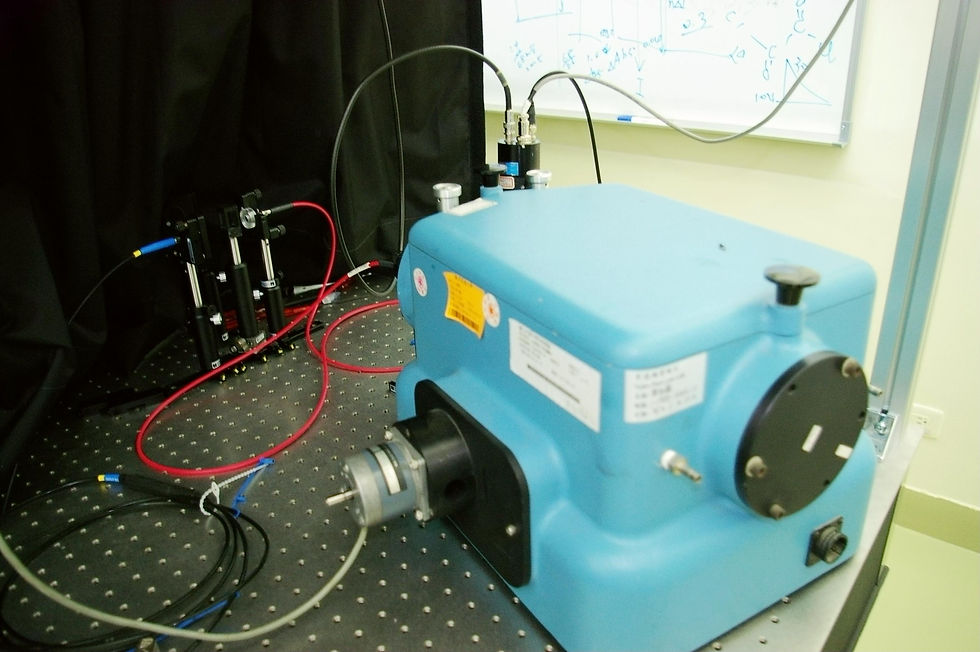
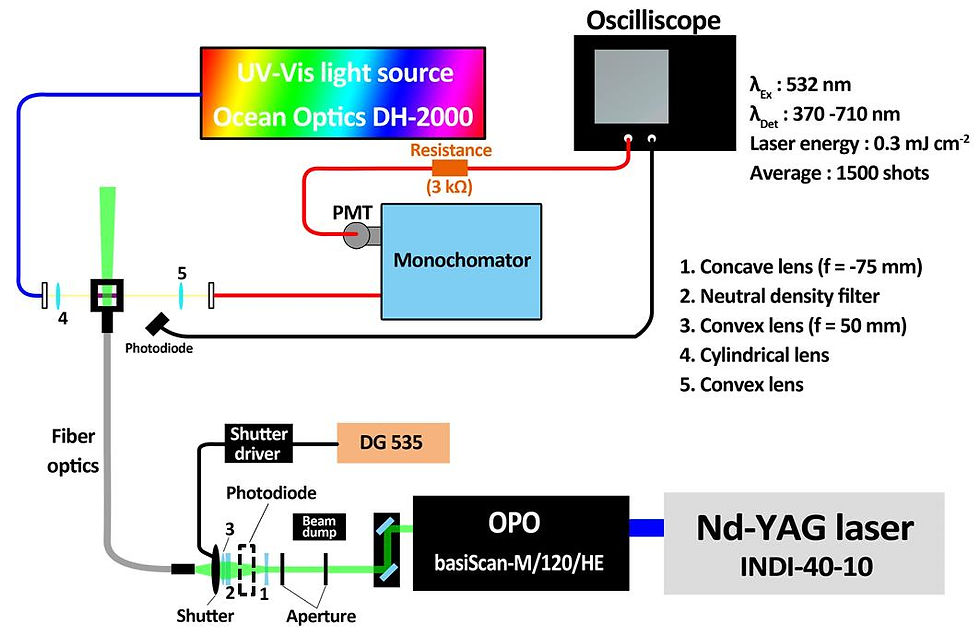
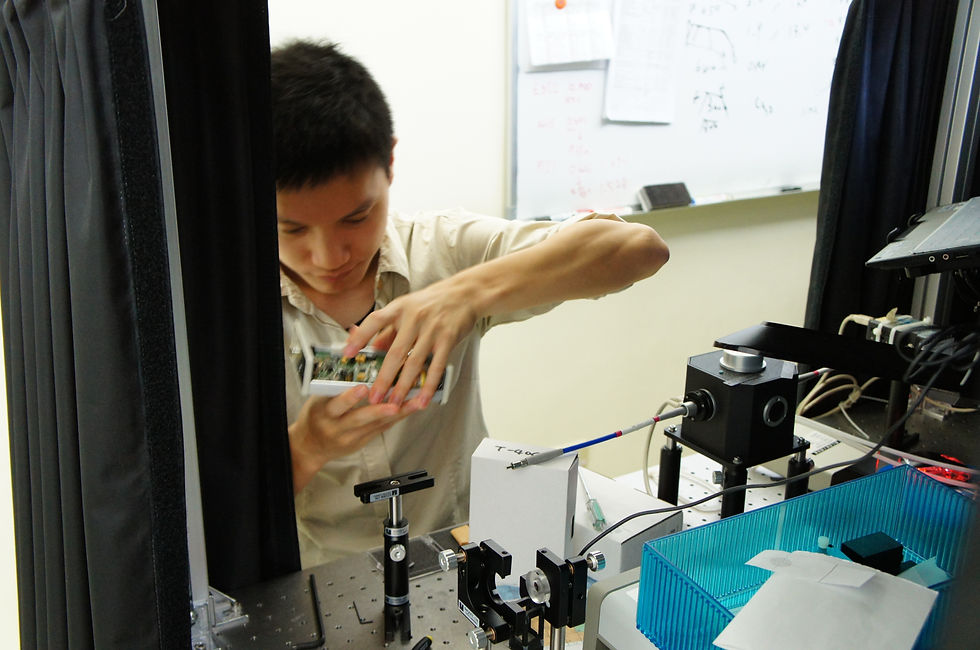

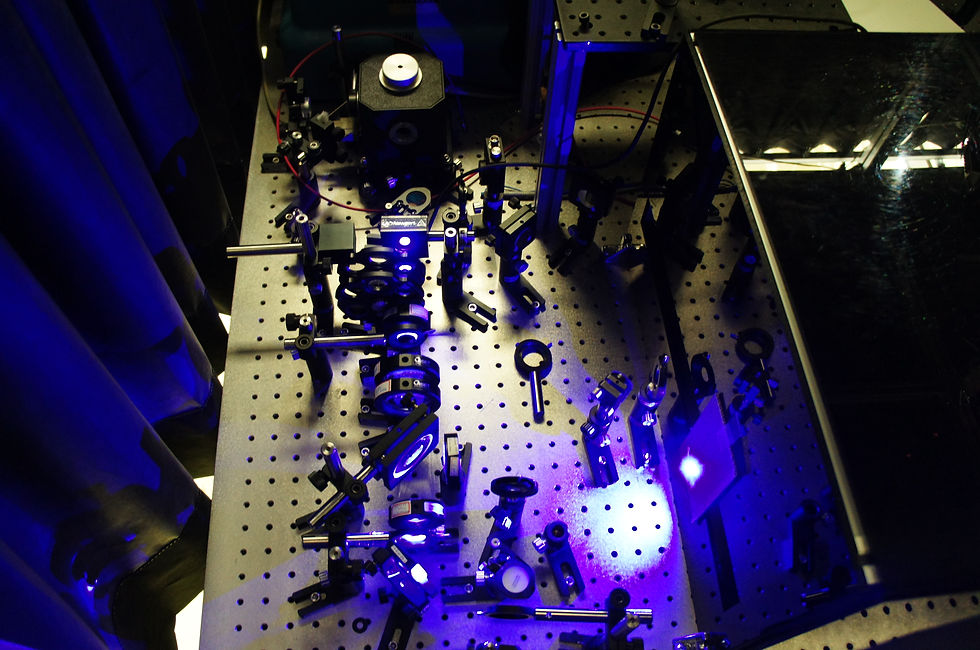

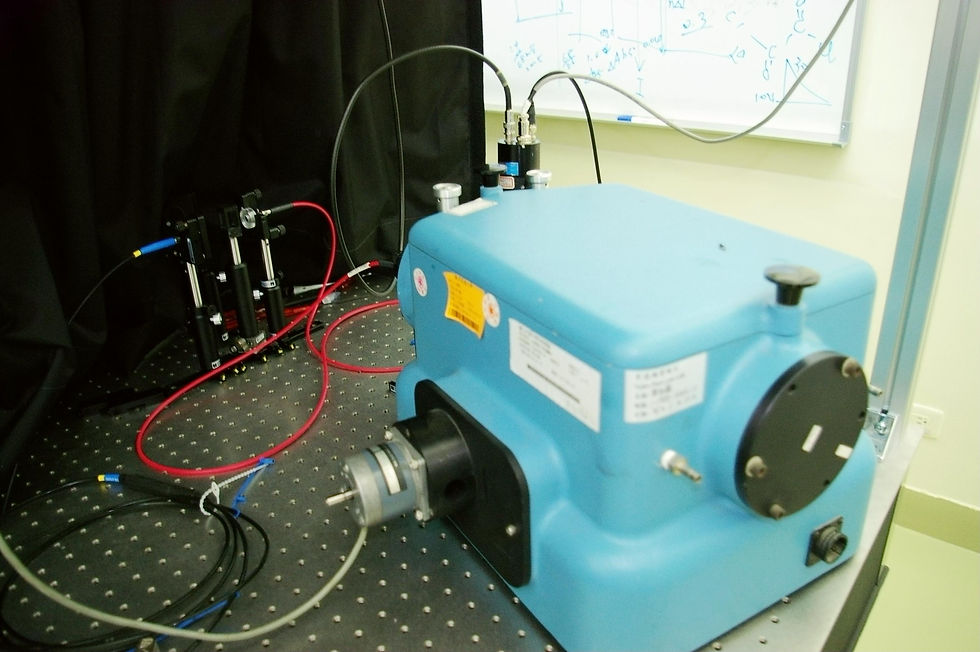
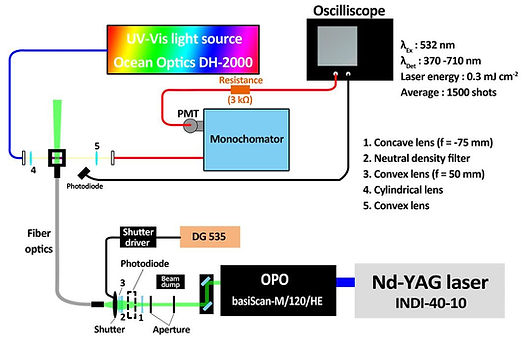
Electrochemical detection module
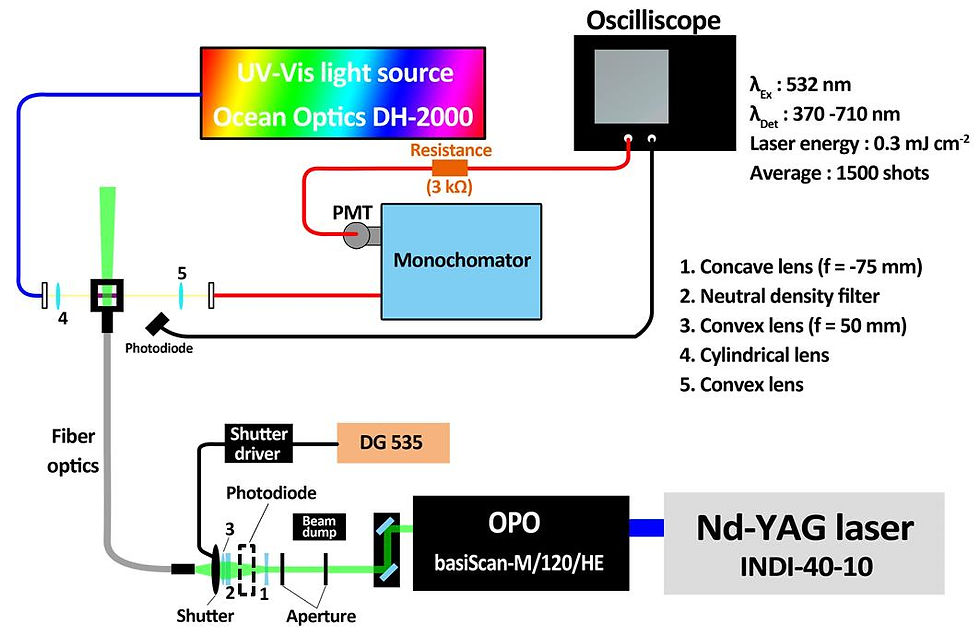
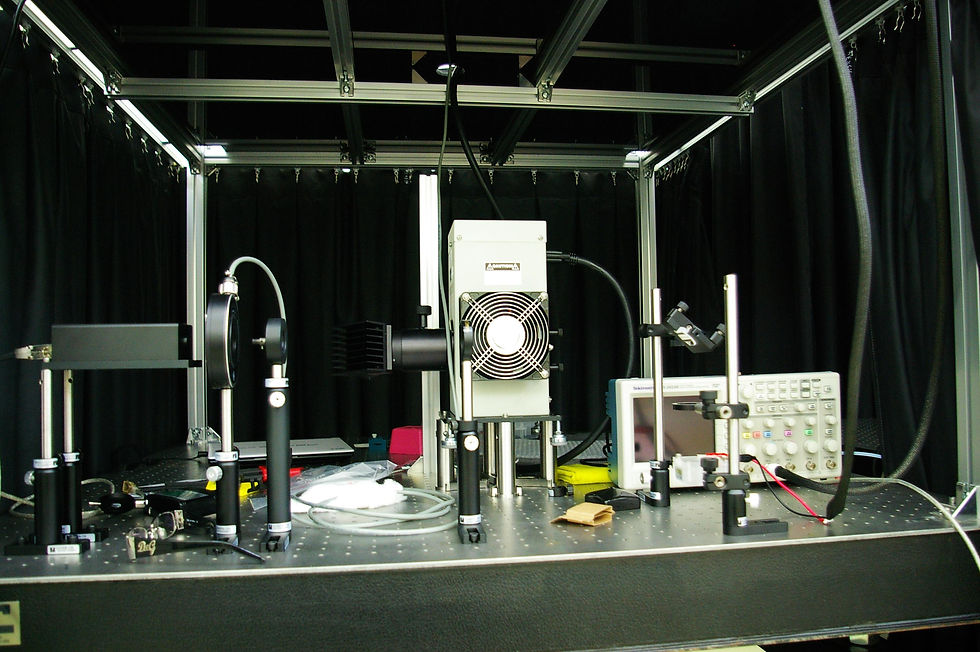

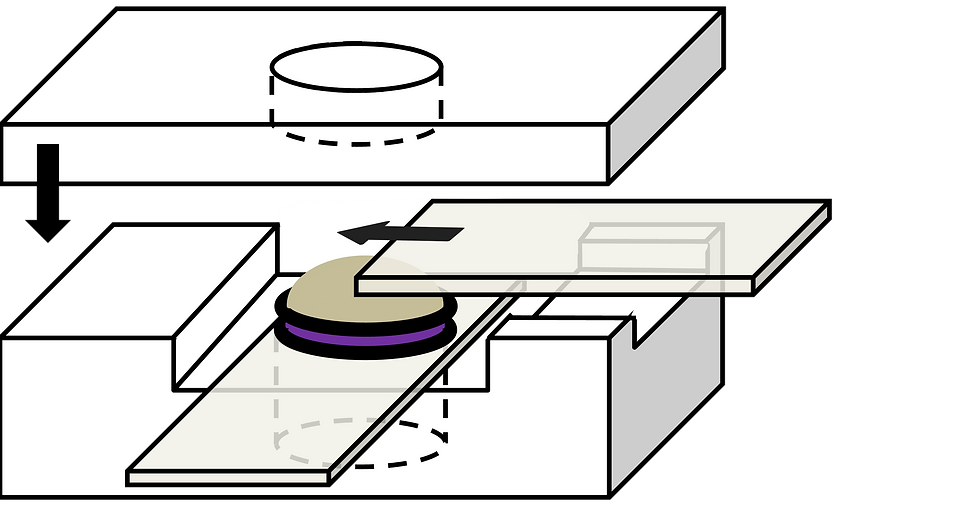
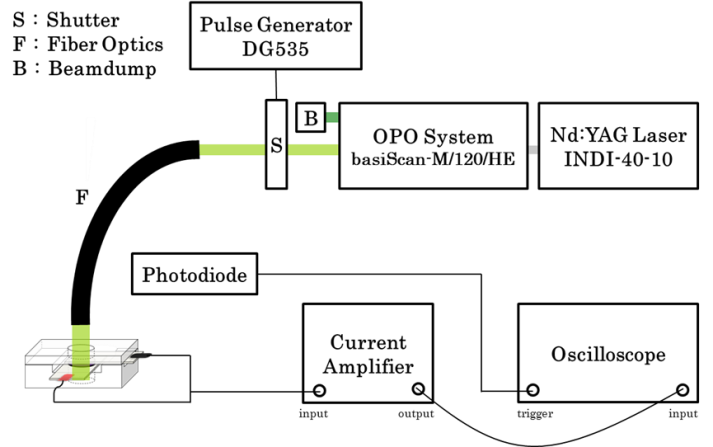
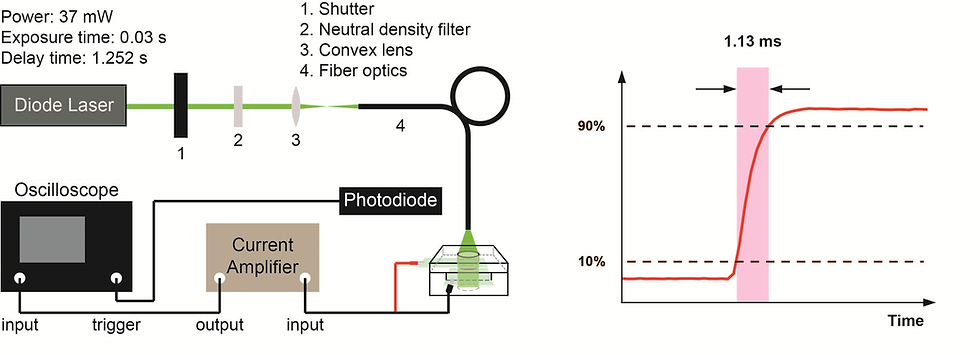
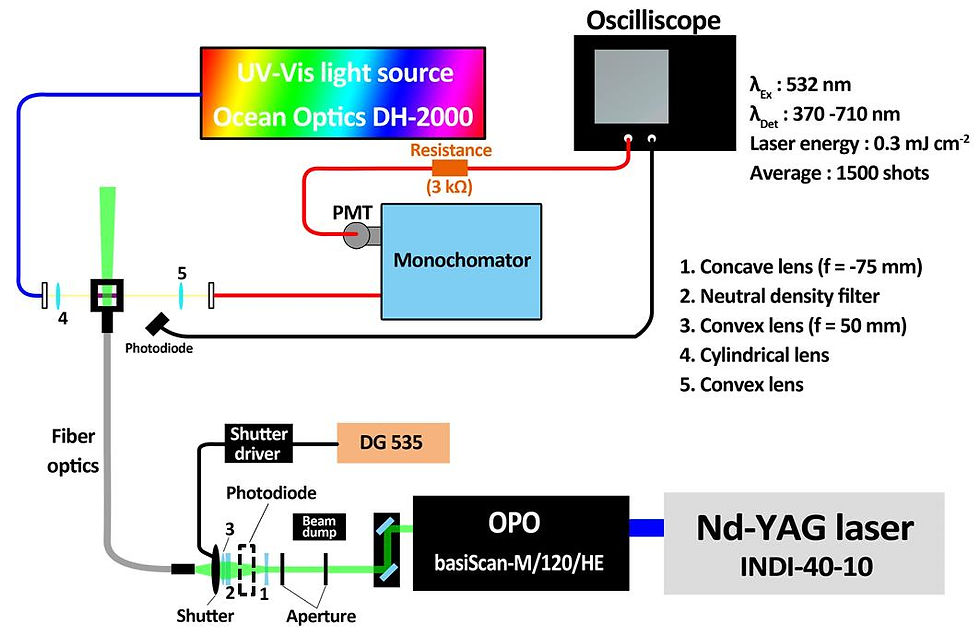
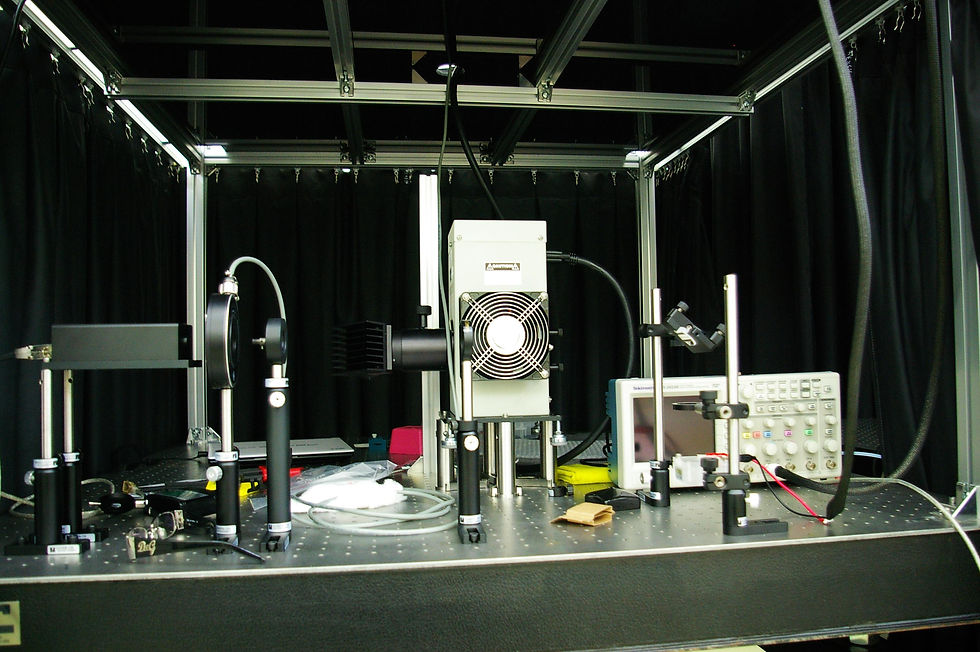

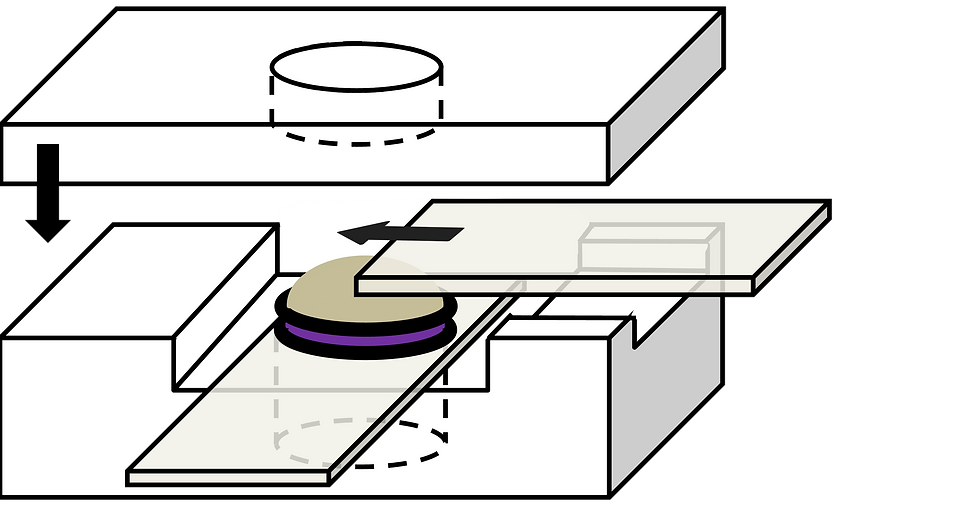
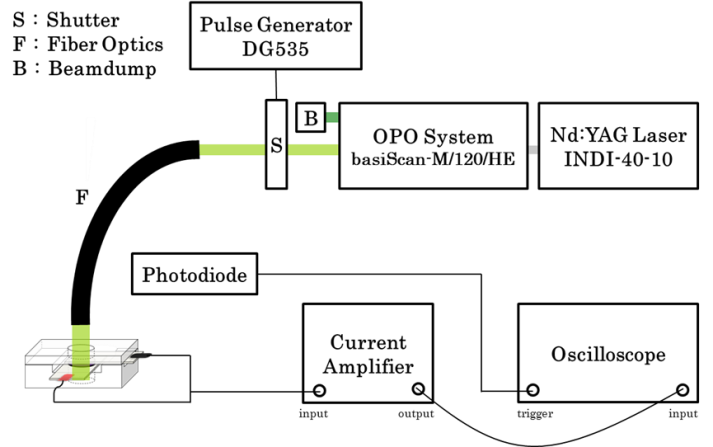
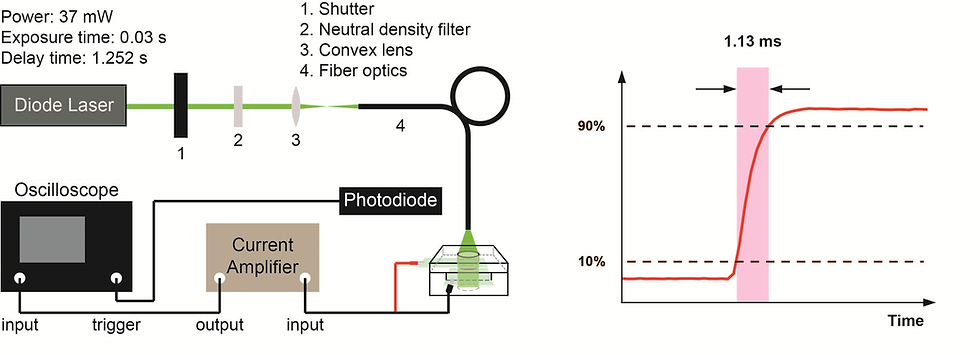
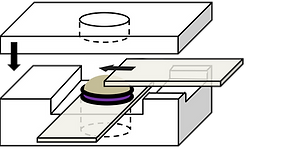

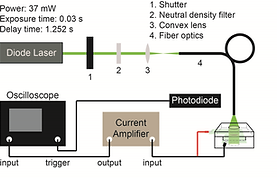
Photoelectrochemical Cell
Electronic components and the solute concentrations were kept identical in working and reference half-cells. Two identical pieces of sodalime glass of 25 mm × 50 mm × 1.1 mm, coated with fluorine-doped tin oxide on a single side, served as the transparent windows and electrodes. A circular Teflon spacer, adhered to a molecular porous membrane on one side, served as the working half-cell and was placed in contact with the FTO surface. The identical Teflon spacer was placed in contact with the reference halfcell and gripped by another coating-up FTO glass.
Pulsed Excitation
An optical parametric oscillator pumped by a frequency-tripled Nd:YAG laser generated the pulsed excitation at 568 nm, with a bandwidth of 3 ns. A mechanical shutter was equipped to decouple the 9.2 Hz irradiation to 2.3 Hz, in order to avoid overshooting. The laser pulse was introduced via fiber optics. The photocurrent was further amplified by an external current amplifier and recorded by a 400-MHz transient digitizer.
CW Excitation
A continuous 532-nm diode laser, modulated by an external shutter with a 30 ms exposure time and 1252 ms full-slew time, served as the modulated continuous excitation source. The excitation beam was further introduced to the front of the electrochemical cell with an optical fiber. The current signal was further amplified by an external current amplifier and recorded by a 400-MHz transient digitizer.